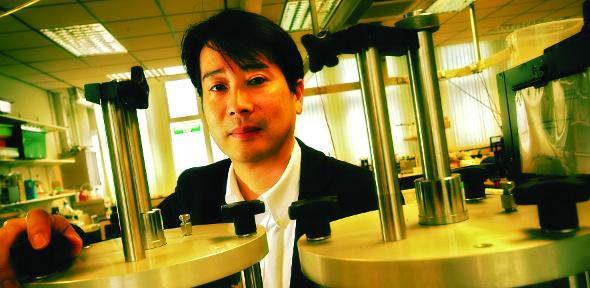
Robert Mair, Abir Al-Tabbaa, Malcolm Bolton, Peter Guthrie, Kenichi Soga and Gopal Madabhushi interviewed by Lydia Wilson, originally in Cambridge Alumni Magazine.
Robert Mair
Robert Mair is Professor of Geotechnical Engineering, Head of Civil and Environmental Engineering, and Master of Jesus College. He specialises in underground construction Most cities in the world are either built adjacent to rivers, on estuaries or on the coast, for obvious historical reasons. That means that the ground conditions are particularly complex because the soils are often soft. As congestion is becoming such a major issue, it is no longer acceptable just to have surface transport. The increasing need for tunnel construction in difficult ground conditions therefore presents major engineering challenges.
In the past, tunnels were only constructed where the ground was known to be strong – the early London Underground, for example, was constructed in strong clay which the Victorians could rely on. But as they pushed further into the south and east of London, tunnels were required in weaker, less stable, ground. If you construct a tunnel in those kind of conditions, it is harder to predict how much the ground will move and what to do to prevent that movement. Even more important is the question of what happens to the buildings above – will they crack, or worse, collapse?
The most famous example of my research in this area was the control of Big Ben’s tilt when the Jubilee Line Extension was being built for London Underground. It was high profile engineering because the Houses of Parliament were watching very carefully.Throughout an intensive 18 month period, Big Ben was continuously monitored with sophisticated instrumentation designed to detect otherwise imperceptible move- ment. Every day crucial decisions were required and small amounts of cement, or grouting, were injected into the ground in a very controlled way deep beneath its foundations. This innovative technique, known as compensation grouting, is now used extensively around the world.
We use our centrifuge at Cambridge for simulating the construction of tunnels by making small scale models and subjecting them to very high gravity; if you get the scaling right it reproduces what actually happens at full size. The centrifuge is very close to my heart, because I did my PhD when it had just been built, and we did some of the very first tests with it on the behaviour of tunnels constructed in soft ground.
There are cities all around the world now realising that they have to go underground to have mass transit systems. I’m advising on the Rome metro, where a new line is being built alongside the Coliseum – it’s every bit as challenging as protecting Big Ben. My research group is also involved in Singapore, where they’re building several new metro systems in ground which can only be described as having the consistency of toothpaste. There are enormous issues about how to build anything safely in such ground, especially large underground structures.
In principle, you can create a whole city underground. Perhaps living there is stretching it, but you can certainly imagine roads, railways, even shopping malls underground. And storage, which can take up a huge amount of space for things like oil or natural gas - why not put them all underground? It of course costs money to create underground space but you can convert a congested polluted city environment to a very green one with only pedestrians and bicycles. There’s no reason why the future couldn’t be underground.
Dr Abir Al-Tabbaa
Abir Al-Tabbaa
Dr Abir Al-Tabbaa is a Reader in Geotechnical Engineering and a Fellow of Sidney Sussex. Her research interests lie in the area of sustainable remediation and regeneration of land from decontamination to re-use of industrial waste.
I have just finished a big project on sustainable brownfield regeneration, which involved a UK consortium of universities and industrial partners. Here in Cambridge we led the work on technical remediation aspects, concentrating on Thames Gateway – the 2012 Olympic site – and East Manchester. The government now gives huge incentives to developers in order to hit their target of 72% of new build on brownfield land by 2016.There is a lot of scope for redevelopment and regeneration, but only if we advance remediation technologies; many techniques are simply not effective enough for some uses of land at the moment.
What I am doing a lot of work on now, which is very exciting, is the research going on into sustainable cement. Concrete is the most widely used building material in the world and millions of tonnes are used every year, using both aggregates – which are quarried, depleting natural resources – and cement. Producing cement is very energy intensive; it is responsible for up to 10% of global CO2 emissions. In the past few decades people have desperately tried to find an alternative for this, but we’re coming from a completely new angle than anything that’s been tried before: a new type of cement.
It is an interesting concept which has been invented and patented by a scientist in Australia. But he has done no experimental work; it has all been from a theoretical chemistry point of view.We are trying to validate his claims by conducting accelerated ageing tests. It’s definitely a potential solution as it works by absorbing carbon dioxide. There has already been a lot of interest; I even have a local building company funding a new PhD student.
Another part of my research is into the future of waste management. Wastes generated from a wide range of industrial processes, for example mining, tunnelling and construction, often end up in landfill, not necessarily because they are contaminated but because they are not suitable for use on the site. I am looking at the conversion of such wastes into beneficial construction products, such as construction blocks, and aggregates which can be used in cement as a replacement for quarried aggregates, thereby reducing landfill as well as the depletion of natural resources. Sustainability issues are moving ahead - but there is still a long way to go.
Professor Malcolm Bolton
Malcolm Bolton
Malcolm Bolton is the Director of the Schofield Centre for Geotechnical Engineering, Professor of Soil Mechanics and a Fellow of Churchill. He describes what is being done to understand the abundant and sustainable, but puzzling, raw material that is soil.
Soil is a natural material, freely available, and although it won’t do everything perfectly – in fact it won’t do many things perfectly – it is very cheap, pliable, long-lasting and almost indestructible. It is also sustainable and recyclable, because when you’re fed-up with your road embankment in one place, you can dig it up and it’s just as good if you compact it and place it somewhere else.So soil is a very neat building material and economical, but it is also weak and very deformable. If you are careless with it, your building foundations will settle and your buildings will crack up; or your railway embankments will creep and you will have to endlessly re-set the rails. All these problems can and do occur, and it’s our job to try and clarify the mechanisms of these aspects of behaviour in a way that graduate engineers will be able to grasp and apply in practice.
However, soil is not easy to test in the lab. Often you get data showing that the soil has peculiar non-linearities and instabilities. One way in which we are trying to understand the nature of soil better is through computer simulations.We represent each soil grain separately, and then subject them, through a computer program, to the laws of Newtonian mechanics. With very few physical parameters given for each grain, the weird non-linear behaviour of soil as a sort of quasi-continuum material actually emerges from the computer simulation. Really, all you’ve got is friction. The fact that you can dig a vertical hole in the ground, or support a heavy building on the ground, that’s all by virtue of friction between separate grains.
But sometimes this can go wrong.Take, for example landslides. If the ground starts moving a little bit more rapidly, the soil seems to lose all its internal cohesion and friction, and even to turn into something more like a fluid. It then can roar downhill and travel a long way down a valley floor, with apparently no friction preventing its motion, taking rocks with it for sometimes tens of kilometres.
I am trying to shed light on the physical origins of this behaviour: how a material which is a granular solid at one moment can turn into something more like a heavy fluid the next second, and then turn back into a solid again; from a sliding mass to a fully mobilised turbulent gas-like flow, and the transition back at the bottom of the mountain. It’s very important: town planners need to know where to put the roads and the developments so that these rock avalanches or debris flows don’t kill people. And it seems at least interesting, and probably useful, to see these numerical solutions and to ponder on what the physics of the problem really is.
Professor Peter Guthrie
Peter Guthrie
In 2000 Peter Guthrie, a Fellow of St Edmund’s, was made the first Professor in Engineering for Sustainable Development in the UK, after spending 30 years as a practising engineer with a geotechnical specialisation
We have been looking at urban development of new communities in the UK because we have a requirement: the government has said that we need 4.4 million new homes. There are issues of sustainability in building new communities, but not so much the things you would expect engineers to look at (how do you make homes more efficient, or use less water, or do sustainable urban drainage) as all of that is pretty well understood now. It is more about how the master plan tackles the really difficult issues of building a community; the interaction of design, social impact, environmental consequences, sustainability and community building.
The master plan is always a vision of a condition that will never exist, because it shows all 10,200 homes and other buildings at exactly the same age and stage, but this is of course not the case: you can only release 500 homes a year, which means it’s a 20 to 30 year process. So dilemmas arise: when you come up with, for example, combined heat and power, do you put in the network of steam pipes for 10,000 homes, or 1000? If you’re putting it in for 10,000 homes, how do you pay for that, when some of it is not going to be drawn on for 30 years? If you do it for 500 or 1000 homes, it usually isn’t economic. So someone has to find within themselves the courage or leadership to make that investment for a nonfinancial return for the sake of sustainability.
For example, we want more use of public transport, and more journeys on foot and cycles. But it’s no good just putting in cycle paths; you have to find out motivators for people to get on a bike, and the de-motivators: poor weather, nowhere to park your bike, lack of security or adequate street lighting, comparison of journey times, where are you allowed to park your car.
You might ask why engineers are involved in this sort of thing, why don’t you just have sociologists or economists or town planners, but there are some very deep technical issues which you need to get to grips with to make the right decisions. For example, there may be a proposal in the early stages to have solar panels on every roof. But it’s probably not going to happen, because solar panel technology is changing all the time. If you design it now and start constructing next year, the technology will be about the same, but if you start constructing in 2 or 5 years it will be completely different, which means you may not want to put solar panels on your roof by then. You have to be careful that the things you decide are not sterilising future opportunities to do something better.
Professor Kenichi Soga
Kenichi Soga
Kenichi Soga is a Professor of Civil Engineering and Fellow of Churchill. His research ranges from the London Underground to contaminants from the cleaning industry, taking in engineering with bacteria along the way.
My main research interest is the monitoring and assessment of infrastructures, especially ageing infrastructures. We work mostly with London Underground, utilising advanced electronics such as fibre optics, wireless sensors, and miniature sensors in an integrated system; now we are installing this wireless sensor network in the Prague Metro, and soon we will do the same for Barcelona.
Many of the structures in the London Underground are large, and many are very old: the oldest was built in the 1860s. They are still going strong, so the question is, how long will they last? I think some will probably go on for many years – in certain places anyway, and as long as the conditions are right.
But if you have a look at the structures built in the 1970s, they are not cast iron, but concrete lined, and there is now degradation of the concrete. We want to know whether that is serious or not. There is a need for constant surveillance, such as our systems will hopefully provide, to tell us when replacement or reinforcement is necessary.
I also head a UK–US research consortium which looks into how we can utilise biotechnology for soil issues. The very first thing we did was to have a workshop, two years ago, to address how engineers can work together with microbiologists, geochemists and environmental engineers to see how we can use bio-soil interactions for specific engineering purposes.
We have a group working on enhancing carbon sequestration of soil, headed by the University of Newcastle. 70% of carbon which is absorbed by plants goes into the soil, where they create carbonates. If you have calcium in the ground this becomes calcium carbonate, which precipitates and then stays in the ground. Many soils can absorb a lot of carbon dioxide in this way, but it’s only in the top 20cm or so of soil. If we can start engineering this process to a deeper level, then we can absorb more carbon.
Another theme under discussion is using bacteria to decompose hazardous chemicals, in a process called in situ bioremediation, which is being headed by the University of Sheffield. By utilising bacteria you don’t need to use as much energy to clean up soils, it is a more sustainable solution if you can harness nature in an appropriate way.
These projects are leading to other engineering applications. The precipitation of calcium carbonate actually binds the particles so that the material becomes stronger. Increasing the strength of the soil may be a good thing when earthquakes happen, or when building railway or road embankments. This binding of the soil also stops water flowing through it by clogging, so we can direct the flow of groundwater.There is a real interest in how bacteria or plants can be utilised for geotechnical engineering in the future.
Dr Gopal Madabhushi
Gopal Madabhushi
Dr Gopal Madabhushi is a Reader in Geotechnical Engineering and a Fellow of Girton. His research ranges from earthquakes and liquefaction of soils to bone and replacement hips, all using the same underlying principles fundamental to engineering.
A major area I am working on is liquefaction of soil. When you have soil particles deposited very rapidly, for example in flash floods, they tend to be quite loose and not well organised. If you get a lot of shaking, for example from an earthquake, the particles want to reorganise themselves into better, denser packing, just like if you shake a bottle of coffee powder, it tends to settle. But sometimes all the gaps are filled with water, for example on a river bed, and during an earthquake there is no time for the water to come out. So the water pressure increases, and sometimes it gets so great that it actually pushes the particles of soil apart, and the particles go into suspension – that’s what liquefaction is.When the soil particles are not in contact with each other any more, and you have a structure on the top, they simply flow out of the way of the structure and allow it to settle as the whole volume of soil is behaving like a liquid.
A potential solution is densifying the soil before building on it, by vibrating the ground. This is all very well if you are constructing a new building or bridge, but what would you do for an old building? Some buildings have been there for several centuries, but are sitting on liquefiable deposits. We have looked at improving the strength of the ground through injecting grout at high pressure into the ground (which then sets), or through biological methods, with microbes cementing the soil particles together, and finally by driving in incline piles, like buttressing. The French are passionate about this, but when we simulated the situation in our centrifuge (using knitting needles) it was only a partial success; the other methods – grout or microbes – seem to work better.
One of the things that is going to change from a land use perspective is the reclamation of land from sea, and the formation of artificial islands, as in Dubai.The technologies we have developed here are hopefully going to play an important role in making the structures on these grounds safe. One way of making new ground is to pump soil in a liquid state and allow it to drain to form an island, which is exactly like the rapid flooding when grains settle in a loose state and are prone to liquefaction. The engineers try to densify it , but that is not so easy with deep soil layers; densification is normally effective only for the top few metres.We can simulate deep deposits in our centrifuge, up to 40 metres thick, and so we are looking at what happens when you have loose deposits with dense deposits on top: what happens to the structure when the foundation soil is liquefied?
In the last 30–40 years, research on superstructures, and how they respond to earthquake loads, has made tremendous progress.That part is much more advanced than where we are, from a foundations point of view. But there is tremendous potential for us to understand. I feel the next couple of decades of earthquake studies will belong to geotechnical engineering.
This article originally appeared in Cambridge Alumni Magazine (CAM) Edition No. 55 Michaelmas 2008.