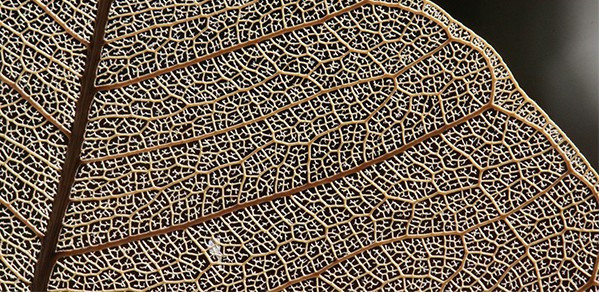
The natural vein structure found within leaves – which has inspired the structural design of porous materials that can maximise mass transfer – could unlock improvements in energy storage, catalysis, and sensing thanks to a new twist on a century-old biophysical law.
We have incorporated this special natural Law into synthetic materials. We hope our work will be important for new generation porous materials and contribute to applications for a sustainable future.
Professor Tawfique Hasan
An international team of researchers, led by the NanoEngineering Group at the Cambridge Graphene Centre, has developed a new materials theory based on ‘Murray’s Law’, applicable to a wide range of next-generation functional materials, with applications in everything from rechargeable batteries to high-performance gas sensors. The findings are reported in the journal Nature Communications.
Murray’s Law, put forward by Cecil D. Murray in 1926, describes how natural vascular structures, such as animal blood vessels and veins in plant leaves, efficiently transport fluids with minimum energy expenditure.
“But whereas this traditional theory works for cylindrical pore structures, it often struggles for synthetic networks with diverse shapes – a bit like trying to fit a square peg into a round hole,” says first author Cambridge PhD student Binghan Zhou.
Dubbed ‘Universal Murray’s Law’, the researchers’ new theory bridges the gap between biological vessels and artificial materials and is expected to benefit energy and environmental applications.
“The original Murray’s Law was formulated by minimising the energy consumption to maintain the laminar flow in blood vessels, but it was unsuited for synthetic materials,” says Binghan Zhou.
“To broaden its applicability to synthetic materials, we expanded this Law by considering the flow resistance in hierarchical channels. Our proposed Universal Murray’s Law works for the pores of any shape and suits all common transfer types, including laminar flow, diffusion, and ionic migration.”
Ranging from daily usage to industrial production, many applications involve ion or mass transfer processes through highly porous materials – applications that could benefit from Universal Murray’s Law, say the researchers.
For instance, when charging or discharging batteries, ions physically move between the electrodes through a porous barrier. Gas sensors rely on the diffusion of gas molecules through porous materials. Chemical industries often use catalytic reactions, involving laminar flow of reactants through catalysts.
“Employing this new biophysical law could greatly reduce the flow resistance in the above processes, boosting overall efficiency,” adds Binghan Zhou.
The researchers proved their theory using graphene aerogel, a material known for its extraordinary porosity. They carefully varied the pore sizes and shapes by controlling the growth of ice crystals within the material. Their experiments showed that the microscopic channels following the newly proposed Universal Murray’s Law offer minimum resistance against fluid flow, while deviations from this Law increase the flow resistance.
“We designed a scaled-down hierarchical model for numerical simulation and found that simple shape changes following the proposed Law indeed reduce the flow resistance,” says co-author Dongfang Liang, Professor of Hydrodynamics at the Department of Engineering.
The team also demonstrated the practical value of Universal Murray’s Law by optimising a porous gas sensor. The sensor, designed in accordance with the Law, shows a significantly faster response compared to sensors following a porous hierarchy, traditionally considered to be highly efficient.
“The only difference between the two structures is a slight variation in shape, showing the power and ease of application of our proposed Law,” says Binghan Zhou.
“We have incorporated this special natural Law into synthetic materials,” adds Tawfique Hasan, Professor of Nanoengineering at the Cambridge Graphene Centre, who led the research. “This could be an important step towards theory-guided structural design of functional porous materials. We hope our work will be important for new generation porous materials and contribute to applications for a sustainable future.”
The research was funded by the Engineering and Physical Sciences Research Council (EPSRC). Professor Hasan is a Fellow of Churchill College, Cambridge.
Reference:
Binghan Zhou et al. ‘Universal Murray’s law for optimised fluid transport in synthetic structures’ . Nature Communications (2024). DOI: 10.1038/s41467-024-47833-0